Mabel
Check out:
all recent videos on our Youtube channel and some old videos have been zipped at the bottom of this page.
​
-
Copyright: These videos and photos belong to me, my colleagues, and/or my students. You may use them for your own ends as long as you cite their source. If you are a MEDIA outlet, contact us for original, unprocessed HD video.
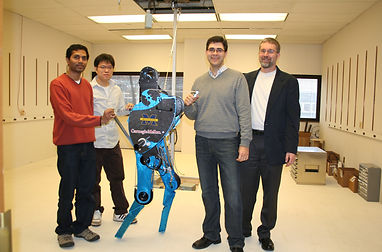
​
MABEL late spring, 2008 (K. Sreenath, H.W. Park, I. Poulakakis, J. Grizzle)
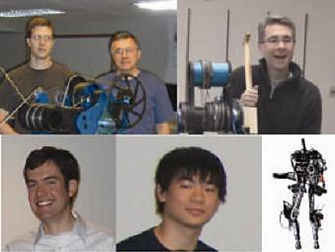
​
Top Row: J. Hurst (CMU), G. Buche (Grenoble), J. Koncsol (GM); Bottom Row: B. Morris (Eaton), S. Zhang (CMU), and RABBIT (Grenoble) all contributed to the test-bed's conception, design, fabrication, and assembly.
MABEL Description
In Summer 2008, we completed assembly of a novel planar bipedal robot for use at the University of Michigan. The robot, named MABEL, was designed in collaboration with Jonathan Hurst, Al Rizzi and Jessica Hodgins of the Robotics Institute, Carnegie Mellon. Jonathan Hurst was responsible for the design of the mechanical systems of the robot. MABEL is comprised of five links assembled to form a torso and two legs with knees. The legs are terminated in point feet. The planar nature of the robot is manifested in the hips, which are constrained to revolute motion in the sagittal plane. The robot is attached to a boom and hence walks or runs in a circle; when the radius is sufficiently large, this corresponds to locomotion along a straight line. Up to this point, the robot is similar to RABBIT, the French robot on which we collaborated from 2001 through 2004.
​
The novel aspects of MABEL, and there are many, appear in the transmission or powertrain . First of all, all of the actuators (four DC-brushless motors, two for each leg) are located in the torso, so that the legs are as light as possible; this is done for running efficiency. In RABBIT and most other robots, the knee actuators are mounted on the thigh. Secondly, the actuated degrees of freedom of each leg do not correspond to the knee and the hip angles (the hip angle being the relative angle between the torso and thigh). Instead, for each leg, a collection of differentials is used to connect two motors (once again, located in the torso) to the hip and knee joints in such a way that one motor controls the angle of the virtual leg consisting of the line connecting the hip to the toe, and the second motor is connected--in series with a spring--in order to control the length (or shape) of the virtual leg.
​
Roughly speaking, the rationale for this design is that it makes the robot a hybrid of RABBIT, a robot that walks extremely well, but never achieved a stable running gait, and a Raibert Hopper, a robot that ``runs'' remarkably well, but can't walk, in part because it has only one leg, but more fundamentally, walking is quite different from bouncing. The springs in MABEL serve to isolate the reflected rotor inertia of the leg-shape motors from the impact forces at leg touchdown and to store energy in the compression phase of a running gait, when the support leg must decelerate the downward motion of the robot's center of mass; the energy stored in the spring can then be used to redirect the center of mass upwards for the subsequent flight phase, when both legs will be off the ground. Both of these properties (shock isolation and energy storage) enhance the energy efficiency of running and reduce overall actuator power requirements. This is also true for walking on flat ground, but to a lesser extent, due to the lower forces at leg impact and the reduced vertical travel of the center of mass. We hypothesize that shock isolation and compliance will be very useful for walking on uneven terrain.
​
The third novelty in MABEL's powertrain is that the springs in series with the leg shape motors are unilateral in the sense that they compress, but do not extend beyond their nominal rest length; instead, once a spring reaches its rest length, the position of the leg-shape motor and the shape of the virtual leg are rigidly connected (i.e., directly, through a gear ratio, and no longer through a compliant element). This is a big advantage in walking where at leg exchange, when the former stance leg must be lifted from the ground, this motion does not have to ``fight'' a spring that is trying to extend due to the non-zero mass of the shin. Similarly, a unilateral spring also makes it easier to initiate take-off in running (i.e., transition from stance phase to flight phase). Roughly speaking, the springs are present when they are useful for shock attenuation and energy storage, and absent when they would be a hinderance for lifting the legs from the ground. The springs can be easily changed from one experiment to another in order to study the relation of spring stiffness to gait efficiency or robustness to perturbations, for example. An optional pre-load is easily established on the springs as well, so that compliance only comes into play when sufficiently large forces are present; this is convenient for testing simplified walking strategies while debugging the electronics and software.
​
The final novelty of the robot is that it is constructed from two monopods joined at the hip. By removing six bolts, half of MABEL's torso and one leg can be removed, yielding a monopod. In fact, Thumper at CMU is literally the left half of MABEL.
​
​
​
MABEL Special Features
Special Features: [Questions by Christine Harbig on June 26, 2007 led me to write up some of the key points of this new machine.] What is the main difference of the new robot when compared to other robots like ASIMO or QRIO? Is it the ability to be able to walk on uneven terrains or mainly a more efficient way of moving? What will be the real step forward in robot technology?
​
There are at least three differences:
-
MACHINE DESIGN: the robot has springs built into the powertrain so that, very roughly speaking, it effectively has the tendons of a kangaroo. This is just to give you a very rough impression. Do not use such a statement in a report! Asimo and Qrio are built of very rigid material and the motors (= power sources) are connected to the joints via rigid gears. In MABEL, the motors for the "knee angles" are connected through springs. Hence, when the robot strikes the ground, the knees flex through the springs, as your foot does when you run in a good sports shoe, as opposed to trying to run in a rigid ski boot. The "hip angles" of MABEL are connected through rigid gearing as in Asimo or Qrio. [technically, we are not using gearing, but instead, pulleys and cables. This is really a technicality to avoid something called backlash that is associated with gears. The main difference is the springs.] Going back to the springs, if you looked at the Raibert hopper, the leg is built from a pneumatic spring, so it hops very nicely. The spring acts like a tendon in an animal. Our robot has roughly the morphology of a human, with the compliance (spring-like character) of the Raibert hopper. It should be able to run very nicely.
-
MOTION CONTROL ALGORITHMS: Asimo and Qrio use a very quasi-static criterion for achieving a stable walking motion. [here, stability refers to the ability to react to perturbations by not falling over, and asymptotically returning to a planned walking or running motion]. These robots use big feet in order to stay upright. The robot Johnnie at the Tech. Univ. of Munich uses the same quasi-static stability notion. In the French robot, RABBIT, a team of us developed a novel theory of DYNAMIC WALKING STABILITY and verified it on the robot. MABEL is more complex, due to the springs, and thus some important extensions must be made to the DYNAMIC STABILITY theory we used for RABBIT. My students, colleagues, and I are making good progress on the design of motion control algorithms forMABEL using an advanced version of dynamic stability. Like RABBIT, MABEL does not really have feet: it will walk and run as if on stilts, the ultimate proof of the dynamic character of its stability notions.
-
SCIENCE PLATFORM: Like RABBIT, MABEL is designed for advancing the science of locomotion and forcing the discovery of more advanced feedback control notions for dynamic motion stabilization. Furthermore, we will soon post the full blueprint of the robot on the web. All of its algorithms are and will continue to be published in leading journals. Students are being trained. Students of all ages are being invited for demonstrations. In short, no aspect of the work is proprietary....everything will be in the public domain. Good luck on learning the details of how Asimo and Qrio function....they are 100% proprietary.
-
Publications detailing the control laws implemented on the robot are available here.
-
A ZIP file providing a symbolic mathematical model of the robot's powertrain is available here. [Right Click and Save]
-
Tons of photographs of the robot's ``insides'' are here.
Our project is very science oriented and very public. This is typical of work funded by the National Science Foundation (NSF) in the US. We are really challenged to push the boundaries of learning.
Back to your question: Is it the ability to be able to walk on different uneven terrains or mainly a more efficient way of moving (smoother movement)? I tried to make out the real step forward in robot technology but I'm not sure about it. The advanced feedback control algorithms and the advanced machine design using springs in the powertrain SHOULD YIELD smoother, more compliant, more energy efficient locomotion. The robot should be able to walk more easily on rough terrain. I say **should** because if we were 100% certain, why build the machine and evaluate the algorithms? There is a very real possibility that we fail.
Noted added on May 24, 2010: It looks like the ability to walk on an uneven surface is now a certainty and not a conjecture! See [YouTube Video, First Attempt at Walking over Rough Ground]. Fast walking has been achieved (we held the speed record from October 29, 2009 through April 2010, and then lost it to PetMan).[YouTube Video, Go MABEL Go!]. High efficiency has been demonstrated; see the efficiency table in the experiment section this paper.
-
​
​
​
MABEL Relevance
Relevance to robots with feet and 3D robots. It is important to understand the relevance of research on planar robots with unactuated point feet in the larger context of 3D robots with feet.
​
Underactuation-101: In a robot with feet, there are phases of a walking gait where the feet are in rotation with respect to the ground, such as toe roll and heel strike, and hence the robot is underactuated (fewer actuators than degrees of freedom). The prevailing technique of control based on the Zero Moment Point (ZMP) can only handle the flat-footed phase, and hence yields very unnatural gaits; moreover, in practice, large (heavy) feet are used to avoid foot rotation. The study of unactuated point feet leads to the development of a control theory for bipeds that deals directly with underactuation. It is then possible to design feedback controllers for robots with feet that yield natural gaits which include both underactuated, fully-actuated and over actuated phases. This becomes even more critical in 3D robots because feet are typically much less wide than they are long, which means that very little torque can be applied in the frontal plane (i.e., side to side) without causing the foot to roll sideways. It is therefore of practical interest to generate a nominally stable gait that requires no ankle torque in the frontal plane, and then use any available torque to enhance the basin of attraction and/or energy efficiency of the gait.
​
Underactuation-201: Once you begin to attack one aspect of underactuation, you are encouraged to try to analyze other aspects. We are now studying underactuation that arises from compliance. This gives rise to higher-dimensional hybrid zero dynamics, a very interesting subject!
​
Generalizations to 3D: We and our colleagues have designed an exponentially stable walking gait for 3D robots with unactuated point feet. This work draws very heavily on the theory of hybrid zero dynamics that we developed for planar walkers; designing the closed-loop system around invariant manifolds and restriction dynamics made the stability analysis much more tractable. Without the theoretical tools systematically developed for planar robots, the analytical complexities present in the 3D problem would have seemed insurmountable.
​
Safety: As a final note, in a laboratory run by faculty and graduate students, without the benefit of a professional technician, a planar robot is safer than a 3D robot; this is because I and my students can easily remain out of the workspace of the robot without enclosing the robot in a cage.
​
​
​
Research objectives
MABEL was designed to be both a robust walker and a fast runner. It pushes the state of the art in bipedal mechanism design and provides an opportunity for effective control design methodology to maximize the robot's energy efficiency, speed, and stability. Our specific objectives are to demonstrate: energy efficient walking on flat ground; the ability to walk over uneven terrain; and running. All of this should be accomplished with provably correct feedback laws. The emphasis on energy efficiency is because, for a biped to be able to operate in the real world for an extended period of time, it must carry its own power and use that power as economically as possible. The ability to walk on uneven ground will allow bipeds to function out of the laboratory. The ability to run is in part for fun and in part to demonstrate dynamic balance in an extremely challenging situation. The running achieved with current bipeds is very unsatisfactory. Just take a look at their gaits and you'll see what we mean. Finally, the emphasis on provably correct feedback laws is because we are attempting to remove a lot of the trial and error from the process of designing control algorithms for bipedal robots. Our objective is to design controllers on the basis of models and have the controllers work on the associated hardware. It's a big challenge! Maybe the biggest one in the list.
​
​
​
Robot Stuff
-
You can download a complete set of CAD files (SolidWorks) for the robot. With these files, you can have all of the parts of the robot machined. Click here then scroll to the bottom of the page.
-
Publications detailing the control laws implemented on the robot are available here.
-
A ZIP file providing a symbolic mathematical model of the robot's powertrain is available here. [Right Click and Save]
-
Tons of photographs of the robot's "insides'' are here.
Videos & Photos
-
The **most current** MABEL videos are now available on YouTube: DynamicLegLocomotion Channel. Because of this, we will update videos on this page less frequently. You can subscribe to the channel for free and have the latest videos at your finger tips!
-
(October, 2009) MABEL is operating nicely. We installed some extra encoders on the robot. The cables in the differential actually stretch more than we ever expected. This causes many problems, as you may expect, if you cannot observe the stretch in your measurements. The additional sensors give us position on motor side and joint side for both knees and hips. Here are some of the cool videos the came out once we improved our measurements.
-
MABEL walking at 1.5 m/s, which equals 3.4 miles per hour. As of the time of this posting, that makes her the fastest bipedal robot in the world in terms of absolute walking speed.
-
Two-legged hopping. This was done to test the accuracy of our dynamic model of MABEL; it's not bad.
-
Compliant Hybrid Zero Dynamics. MABEL walks with the mathematical muscle of the hybrid zero dynamics!
-
-
(July 9, 2009) Back on line and operating. We were down with power amplifier problems and a number of other hardware issues. We seem to have them resolved. We also conducted parameter identification for the robot and hence we now have a good model for determining (near) optimal trajectories. The pre-loads on the springs have been set to zero. The controllers used in the videos below employ virtual constraints determined from our analytical model. The resulting gaits are MUCH nicer than the gaits obtained with virtual constraints designed by hand. They are also twice as energy efficient. Here, the virtual constraints are still implemented via high-gain PD control.
-
MABEL walking at 0.9 m/s taking advantage of the compliance. As stated above, the gait is twice as efficient as a similar gait designed by hand. As has been said many times before, there is nothing more practical than a good theory!
-
Robustness test. MABEL gets pushed around and keeps on walking.
-
Slow Walking. MABEL's torso is leaned backward by an increasing amount, while all other aspects of the gait remain unchanged, until the robot can no longer continue walking and falls. It is surprising how far back the torso can be leaned.
-
Hand-designed virtual constraints. This video is from August 8, 2008. Compare the gait here to any of the gaits in the above newer videos. In addition to not looking pretty, it is less energy efficient by a factor of two.
-
-
(August 8, 2008) A few nice laps to show that the robot is functional. We programmed a controller using hand-tuned virtual constraints because we have not finished identifying the center of mass and inertia parameters of the robot. For the first video, pre-tensions on the springs were set, effectively removing the springs from the powertrain. In the second video, the pre-tensions are removed, so the springs are absorbing the impact forces. They make a significant difference. Note that a "falling" video is included to show that the bar does not hold up the robot. This incident occurred when we inadvertently severed a communication cable.
-
Walking at 1.2 m/s, without taking advantage of the compliance: High Def for PC (Big but worth it at 148.6MB) and MAC (12.7MB)
-
Walking at 0.98 m/s, this time taking advantage of the compliance High Def for PC (Big but worth it at 157MB) and MAC (13.4MB)
-
Falling due to a severed cable PC (8MB)
-
-
(November 2007) The construction of the bipedal test bed included several phases. The videos below show the assembly process of the robot itself, once fabrication was complete. The videos are meant to be fun; they are not all that informative! The fabrication of the electronics and the composition of the code for the real-time control system are less visual and are not shown. Those parts took a long time. The videos are only posted in a form compatible for PCs (.wmv).
-
Photos (the files are large; you should right click and save to your hard drive before opening them)
​
​
​